Abstract
Silver is an effective germ fighter and silver nanoparticles are widely recognized as being especially effective because of their enormously high surface area. Due to a large number of manufacturers using silver nanoparticles in their products, some concern has arisen about the effects on the environment when these products are disposed of or washed.
This report will demonstrate that silver nanoparticles do not remain “nanosize” when they come in contact with normal environmental samples, such as soil and water, but they agglomerate to form much larger, much less biologically effective, silver particles which are non-toxic, non-ionic and have no history of being harmful to the environment or aquatic life. Furthermore, there is no possibility that silver nanoparticles can ever form silver ions, except in the presence of strong oxidizing substances.
Introduction
Recently, the Environmental Protection Agency (EPA) issued a statement that they were planning to regulate companies that produce nanoparticles for use as antimicrobials. This gives rise to the question: why are the dietary supplement and nanoparticle industries being targeted at this time and what is the rationale for new regulation of an industry which has previously had no reported harmful effects to humanity or the environment?
The EPA knows that silver nanoparticles are effective as antimicrobials. The reason given by the EPA for the current interest is that silver nanoparticles, or products that claim to be silver nanoparticles, are now being produced by a number of manufacturers, and the EPA is concerned that, when these particles are disposed of, there might be an appreciable amount of silver nanoparticles suddenly appearing in the environment.
The proposed concern is due to the fact that the silver nanoparticles are so small that their surface area per unit weight is very large, therefore, for a given weight of the product, the biological effectiveness, which is proportional to the surface area, is far beyond that which would be expected. This much is true and it is part of the reason that silver nanoparticles are so attractive in biological applications. The EPA is not questioning the fact that silver nanoparticles are effective in killing harmful bacteria, but that, because of the high surface area/weight effectiveness parameter, by disposing these particles into sewers or waterways, might there be harmful effects to the environment by eliminating the bacteria which are useful in normal waste degradation?
The last statement shows a misunderstanding of what silver nanoparticles are and what they do. Nanoparticle technology is relatively new to the scientific community for good reasons: Nanoparticles are difficult to produce; they are difficult to stabilize once they have been produced; they are not stable enough to exist in nature for very long. The purpose of this work is to prove that normal interaction of nanoparticles with various soils and different water sources is sufficient to change the size and dramatically decrease the biological activity.
Specifically, the areas of the examination will include experiments which will establish that silver colloids, which start out as nanoparticles, upon contact with the environment “grow” to much larger clusters, as indicated by their average particle size distribution, (a nanoparticle size measurement), and zeta potential measurements, which will establish that the zeta potential is outside of the range required for nanoparticle stability.
There are several other areas which, as recent articles have indicated, show misunderstandings about silver and its nanoparticles. At Arizona State University, Westerhoff and Benn (1) have reported “findings” which have never been observed during the last 10 years at Colloidal Science Laboratories (CSL). For example, they claim that nanosilver particles produce ionic silver when exposed to moisture. This is NOT true! This is tantamount to saying that silver metal is water soluble.
At CSL, various forms of silver, ranging from solid silver metal to fine silver powder to colloidal nanoparticles, have been exposed to water for long periods of time with agitation. No increase in conductivity or silver ion concentration has ever been observed when the silver metal in any form is treated with water. Silver metal requires chemical treatment with nitric acid or Aqua Regia to make silver ions.
Westerhoff and Benn were working with commercially prepared socks which are prepared by treating the socks with solutions of silver in questionable form. There may have been some actual colloidal silver in the treatment solutions, but there most certainly were soluble silver compounds present, and, once the socks are rinsed, these are the substances which put silver ions back into the wash water.
Some people also claim that only silver ions have antimicrobial properties. This is another misconception. Colloidal silver is a wonderful antimicrobial by itself, which is a good thing, because silver ions are very reactive with chloride ion to form insoluble, and biologically inert, silver chloride. This happens in the bloodstream and in nature wherever halogen anions are present.
As this report will show, the high biological effectiveness of colloidal silver does not persist in nature because the nanoparticles agglomerate as soon as they come in contact with the environment, specifically soil and water. Westerhoff and Benn admit that silver particles “clump” together in the fabric and in the wash water. That is precisely the point to be considered for environmental safety. How much “clumping” does it take so that the particles are no longer considered to be “nano”, but much larger, therefore eliminating the continuing effect of high biological activity?
These researchers and others are very quick to make the jump from colloidal nanoparticles, in socks for example, to ionic silver and its toxic effect, especially in zebrafish, and they speak as if the ions come from the colloidal nanoparticles. It is necessary to be very clear about this. If the researchers are finding silver ions in the wash water or anywhere else, then the silver ions were present in the original material. This cannot be stated too strongly. Unless people have taken to washing their socks in nitric acid, the conversion of colloidal silver nanoparticles to silver ions is not possible.
On the other hand, it is not surprising that certain research institutions are being encouraged, and perhaps even funded, to conduct research that implies toxicity for colloidal silver. According to well-known internet health forum host, Tony Isaacs, the reason for this is that “The only thing colloidal silver is toxic for is the profits of big drug companies.” Mr. Isaacs also states “Silver has been used effectively by mankind to fight germs and ailments for thousands of years.” “It (colloidal silver) is far safer, more effective and less expensive than the marginally effective and side effect laden mainstream antibiotics.” (2)
The work at hand will examine four different environmental conditions which change the morphology and stability of silver colloids:
- The effect of drainage of silver colloids through several soil samples. 2. The effect of the interaction of silver colloids with different water samples.
- The effect of exposure of silver colloids to sunlight.
- The change in the level of silver colloids with regard to biological activity.
Experimental
Sample Selection
At the outset, the first two questions to be addressed were what environmental samples should be used and to what concentration of colloidal silver should these samples be exposed. Since this is the first attempt at this kind of information, it was decided to limit the environmental samples to the following:
- Sand, taken from the New Jersey shore 2. Dirt, taken from central New Jersey,
- Dirt, taken from Northern Pennsylvania
- Water, local tap water from Westampton, NJ
- Water, seawater, taken from the New Jersey shore 6. Water, taken from a northern Pennsylvania well
The soil samples represent some of the most common types found on the Eastern Coast of the United States. The sand is essentially an Entisol, which is a type of soil that is not subject to a great deal of chemical change and is common to areas where deposition and removal occur at regular intervals. The New Jersey soil is primarily an Ultisol, which contains clay, quartz, kaolinite and various iron oxides. The Pennsylvania soil is most likely a mixture of Alfisols and Inceptisols which are clays that are productive for growing most crops and are common to many areas. (3)
The water samples are Sea water, rich in many salts, NJ tap water, which has been through routine purification, and Pennsylvania well water, which most likely contains carbonates and nitrates. Therefore, the selection of samples should be sufficient to establish the effect of the environment on nanoparticles for this initial study.
Approximately 8 to 10 lbs of each environmental sample were collected. From these, 18 to 20 samples of 20.0 g each were selected, and these were randomized for the testing.
Next, in looking at the quantitative amount of colloidal silver to be used, it was decided that the initial test case should provide information with regard to an overabundance of nanoparticles being released to the environment, rather than just a trace amount. If the environment is not substantially altered by the overabundance, surely it will not be influenced by smaller amounts.
Preliminary work indicated that, at concentrations of up to 6 ppm, and probably higher, based on the weight of soil samples, no nanoparticles would survive. Therefore, a more reasonable amount, but still an enormously high concentration for a natural occurrence, was selected.
Colloidal silver samples at our disposal were of the dietary supplement type and average at least 20 ppm of silver. Most soil samples require 0.5 to 0.75 their weight in water to start draining. It was decided that the colloidal silver would be diluted 10 to 1 and then applied to each soil sample. This would make each sample contain a minimum of 2 ppm of silver nanoparticles, based on the weight of the soil. This would correspond roughly to dumping 27 liters of 20 ppm colloidal silver onto one ton of dirt. Since most colloidal silver customers are concerned with teaspoon and tablespoon quantities, it should be safe to say that this experiment covers something well above the worst case scenario.
Measurements
In each experimental case, the selected sample of colloidal silver was mixed with the environmental sample and the change in particle size and zeta potential recorded after a specified time. The instrument used for this work was the Malvern Zetasizer, Model Nano ZS. Since the samples which were in contact with soil contained very large macroparticles and rocks, the samples all required vacuum filtration through grade 601 Ahlstrom filter paper to eliminate the particles which are 3 to 4 orders of magnitude greater than the ones of interest in this study. This filtration has no effect on nanoparticles.
For the trials in which the environmental samples were water, the colloidal silver was diluted 10 to 1 in the water in question.
Results
The initial data in this section shows the properties of the colloidal silver used in these trials. This sample, selected at random, had 81% of its particles at 1.74 nm, and a Zeta potential of -31.7 mV. The data in Tables 1 through 6 show the results of the particles found in the fluid after the specified time of contact with the environmental samples in question. For example, it is shown in Table 1 that when DI water was filtered through the soil samples, no nanoparticles could be found, but only large particles on the order of 300 nm or more.
Table 2 shows that, after only 15 minutes of contact with the soil samples, a decrease in zeta potential, and the smallest particles have increased to the 3 to 8 nm range, and they still represent 80 to 90% of the total.
Table 3 indicates that, after a full 7 days of contact with the soil, but kept away from sunlight, the nanoparticles have increased 3 to 8 times in size.
In Table 4, these results are more dramatic, since the samples were all exposed to the sunlight for the 7 days, with the increases in size being 7 to 20 fold, and the smallest particles now representing only 30 to 40 % of the total.
For the data in Table 5, the colloidal silver was left in contact with the environmental water sample for 21 days in sunlight. As can be seen from the table, the particle sizes have significantly increased (3 orders of magnitude), with a corresponding drop in the zeta potential.
In Table 6, the samples were left in contact with the water samples instead of the soil samples for 7 days in the sunlight. The results of these tests show that each water sample also decreased the zeta potential and increased the particle size.
PROPERTIES OF COLLOIDAL SILVER USED IN TESTING
Smallest Particles, nm | Zeta Pot., mV | Total Ag, ppm | Ionic Ag, ppm |
1.74 | -31.7 | 21.4 | 9.60 |
TABLE 1 – DEIONIZED WATER
Filtering Medium | Smallest Particles, nm | Zeta Pot., mV | Total Ag, ppm | Ionic Ag, ppm |
Sand | None found | -20.2 | 0.00 | 0.00 |
NJ Soil | None found | -1.5 | 0.00 | 0.00 |
PA Soil | None found | -31.3 | 0.00 | 0.00 |
TABLE 2 – COLLOIDAL SILVER – 15 MIN. CONTACT – 7 DAYS LATER
Filtering Medium | Smallest Particles, nm | Zeta Pot., mV | Total Ag, ppm | Ionic Ag, ppm |
Sand | 3.53 | -20.6 | 1.14 | 0.00 |
NJ Soil | 4.35 | -22.2 | 1.57 | 0.20 |
PA Soil | 8.30 | -21.7 | 1.05 | 0.20 |
TABLE 3 – COLLOIDAL SILVER – 7 DAYS CONTACT – NO SUNLIGHT
Filtering Medium | Smallest Particles, nm | Zeta Pot., mV | Total Ag, ppm | Ionic Ag, ppm |
Sand | 5.4 | -15.7 | 1.27 | 0.00 |
NJ Soil | 9.7 | -20.8 | 0.56 | 0.00 |
PA Soil | 14.7 | -2.8 | 0.17 | 0.00 |
TABLE 4 – COLLOIDAL SILVER – 7 DAYS CONTACT – SUNLIGHT
Filtering Medium | Smallest Particles, nm | Zeta Pot., mV | Total Ag, ppm | Ionic Ag, ppm |
Sand | 11.3 | -22.8 | 0.94 | 0.00 |
NJ Soil | 26.9 | -22.2 | 0.41 | 0.00 |
PA Soil | 34.2 | -21.2 | 0.35 | 0.00 |
TABLE 5 – COLLOIDAL SILVER – 21 DAYS CONTACT – SUNLIGHT
Filtering Medium | Smallest Particles, nm | Zeta Pot., mV | Total Ag, ppm | Ionic Ag, ppm |
Sand | >2000 | -11.3 | 0.03 | 0.00 |
NJ Soil | >1900 | -4.6 | 0.24 | 0.00 |
PA Soil | >1700 | -7.6 | 0.39 | 0.00 |
TABLE 6 – COLLOIDAL SILVER – 7 DAYS CONTACT
Filtering Medium | Smallest Particles, nm | Zeta Pot., mV | Total Ag, ppm | Ionic Ag, ppm |
Tap Water | 113 | -11.3 | 0.03 | 0.00 |
Sea Water | 631 | -4.6 | 1.14 | 0.00 |
Well Water | 32.1 | -15.7 | 1.47 | 0.20 |
While some of the changes in particle size seem small, one must realize that they represent large changes in loss of surface area and, since biological activity is proportional to surface area, this would correspond to large losses in biological effectiveness. In Figure 1, it can be seen that a change in particle size from 2 to 10 nm represents about an 80% loss in surface area for the same weight of particles. This is an approximation since the exact morphology of the particles is not known. To make these calculations possible, an assumption has to be made that the particles are spherical and the spheres are close packed.
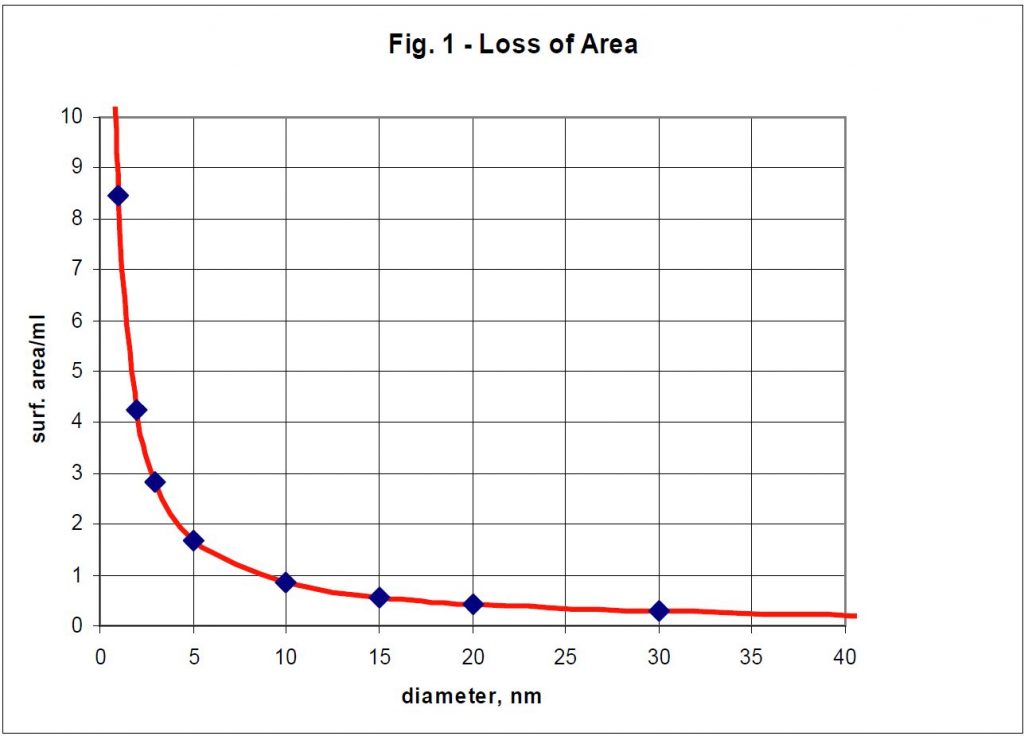
In a previous paper by F. Key and G. Maass (4), the nature of a colloid was described as being a suspension of very small particles which are stabilized by having a diffuse double layer of solution ions around them. The charge acquired by these particles gives rise to a potential difference (i.e., mutual repulsion) between them that keeps them separate and stabilizes the colloid. This potential difference is called the Zeta Potential, and has been described in countless books on electrolytic effects in solutions. When the colloid is composed of nanoparticles, the task of preventing the agglomeration is not an easy one.
As the previous paper pointed out, if the zeta potential is more negative than-30 mV, then the mutual repulsion between particles is sufficient to keep them separate and stabilize the colloid. When the zeta potential is between -15 mV and 0 mV, however, the particles agglomerate and flocculation or precipitation occurs.
In a 1996 report by the Department of the Interior by M. Elimelech and A. E. Childress (5), it was pointed out that for world average fresh water rivers, the concentration of common anions and cations across all normal pH ranges is sufficient to change the zeta potential range from about -10 mV to +5 mV, making agglomeration of nanoparticles occur. In sea water, the agglomeration would be even more pronounced.
Conclusions
Theoretically, if a very large amount of silver nanoparticles from many sources were to be dispersed into the same part of the environment at the same time, it might be
possible that the concentration of some good bacteria, as well as the bad bacteria, would be diminished, but this is not at this time considered a serious threat for the dietary supplement nanoparticles. The points to be remembered are as follows:
- This report has demonstrated that silver nanoparticles will grow to biologically far less active “clumps” even if one dumps 27 liters of 20 ppm colloidal silver on each ton of soil. In practice, this is an enormously high number which could not be expected to be reached realistically.
- In spite of the number of manufacturers producing silver nanoparticles or claiming to be silver nanoparticles, because of the low concentrations in which these products are sold, the total amount which could be released in any part of the environment would still be expected to be very low.
- As shown by all the experiments above, nanoparticles do not last as nanoparticles in nature for very long, but grow to harmless clumps of silver metal.
- Silver nanoparticles are not water soluble, and therefore, silver colloids will not release silver ions into the environment.
Once agglomeration of the silver nanoparticles occurs, the result is simply silver metal; a harmless metal which has existed in nature from the beginning of our planet. Most people would not object to finding silver metal on their property.
References
- Environ. Sci. & Technol. 2008, 42, p 7025-7026
- Tony M. Isaacs, www.americanchronicle.com/articles49272, May 14, 2008, 3. Dept. of Agriculture, Handbook 296, 2006
- F. Key and G. Maass, “Ions, Atoms and Charged Particles”, available at Silver Colloids publications
- Zeta Potential of RO Membranes by M. Elimelech and A. E. Childress, contract No. 1425-4-CR-81-19290
ABOUT THE AUTHOR
Dr. George Maass is the chief chemist for Colloidal Science Laboratories and serves as senior scientific advisor to Purest Colloids, Inc. He holds a BS in chemistry from Fordham University and a Ph.D. in physical chemistry from Iowa State University.
For the last 12 years, Dr. Maass has been an adjunct professor of chemistry at Camden County College, while operating his own consulting business. He has authored papers and presented seminars on his work in the all across the US, as well as in England and in Mexico.
Dr. Maass, a recognized problem solver, has the ability to determine the facts which cause phenomena, and to determine the methods by which they can be controlled.
© 2008 Colloidal Science Lab., Inc.